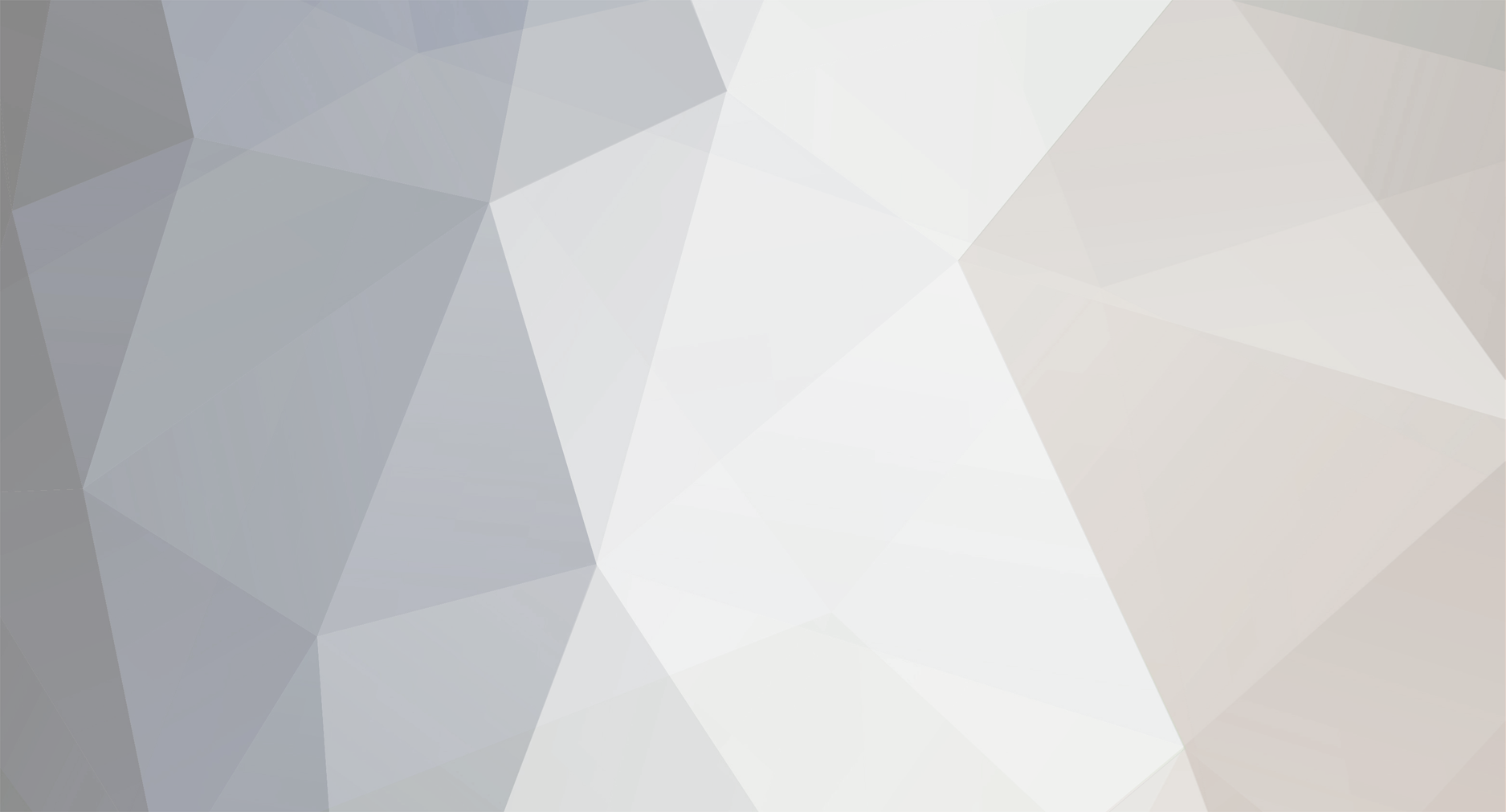
Exhemus
-
Брой отговори
1932 -
Регистрация
-
Последен вход
-
Days Won
10
"Форум Наука" е онлайн и поддържа научни, исторически и любопитни дискусии с учени, експерти, любители, учители и ученици.
За своята близо двайсет годишна история "Форум Наука" се утвърди като мост между тези, които знаят и тези, които искат да знаят. Всеки ден тук влизат хиляди, които търсят своя отговор. Форумът е богат да информация и безкрайни дискусии по различни въпроси.
Подкрепи съществуването на форумa - направи дарение:
Дори малко дарение от 5-10 лева от всеки, който намира форума за полезен, би направило огромна разлика. Това не е просто финансова подкрепа - това е вашият начин да кажете "Да, този форум е важен за мен и искам да продължи да съществува". Заедно можем да осигурим бъдещето на това специално място за споделяне на научни знания и идеи.